Download this chapter for offline viewing (no videos, 3MB)
Download this chapter for offline viewing (includes video content, 127MB)
After the studies of Edwin Hubble revealed that the Universe was expanding, cosmology leapt forward to the Big Bang theory and its elegant explanation of how the Universe came to be. In recent years the combination of increasingly accurate observations and the need to merge the probabilities of quantum mechanics to the certainty of General Relativity led to a series of new theories that have been attached to the initial Big Bang hypothesis. As a consequence, the originally simple theory has been patched by a series of disparate triage theories to form what has become the current paradigm for modern cosmology.
Historic Paradigm Shift
Eratosthenes a Greek mathematician, geographer and astronomer, who was appointed librarian of Alexandria in 236 BC, around 255 BC invented the armillary sphere. The sphere is a model of the objects in the sky centered on the Earth with their positions in celestial longitude and latitude. The armillary sphere established the ecliptic where the solar system objects moved through a background of stars. Note that Eratosthenes represented the Earth as a round sphere.
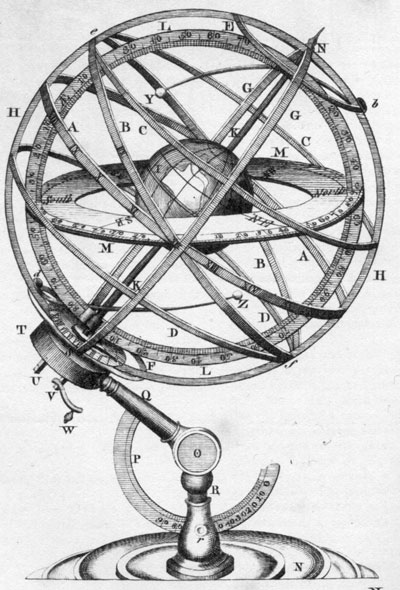
Around 240 BC he determined the Earth’s circumference in an experiment measuring the difference in the angles of the shadows for a standardized measuring stick at noon on the summer solstice. One stick was placed in Syene, now Aswan, Egypt and the other was in Alexandria. Syene was located on the Tropic of Cancer, and therefore showed no shadow. While the angle of the shadow in Alexandria (7º) indicated the difference in the curvature of the Earth between these points. From this he estimated, quite accurately, the circumference of the Earth.
Claudius Ptolemy (c. AD 90 – c. AD 168) a Greco-Roman mathematician, geographer, and astronomer of Alexandria developed astronomical models, which predicted future and past positions of planets. His models were geocentric since the Earth was considered to be the center of the universe. At the time, this was an elegant solution to observations without the aid of telescopes. His charts and predictions of the movement of the planets, Sun and Moon helped his geocentric model become the accepted paradigm for the structure of the universe.
Nicolaus Copernicus (1473 – 1543) developed the idea that the Sun was the center of the Universe and in his manuscript of 1514 published his conclusions. His heliocentric model was a more elegant explanation for the movement of the astronomical objects than the Ptolemaic model. His theory gradually became a subject of much debate.
Galileo Galilei (1564 – 1642) after some initial work done by spectacle makers in the Netherlands developed what became the first effective telescopes. The increasingly accurate observations made with these instruments made it progressively more difficult to explain various observed phenomena with the geocentric model. This is apparent in geocentric viewpoint in Kepler’s drawings of Mars’ movements “around the Earth”.
Ptolemy’s model had been the basis of astronomical philosophy for 1,400 years. Its sacrosanct Earth-centered philosophy was adopted by the church and became a justification for religious persecution, as Galileo was to find out. The increasingly subtle observed movements required progressively more complex explanations under the Ptolemaic model, ultimately making its explanation untenable.
However, the Copernican model survived the slow but inevitable increased scrutiny that followed. Copernicus replaced the geocentric- with the heliocentric-universe resolving in one simple stroke the unexplained intricacies of the former model. These complexities were swept away by Copernicus’ heliocentric paradigm shift.
This shift opened the way for Johannes Kepler’s (1571 – 1630) who, using the extraordinarily detailed mapping of the heavens by Tycho Brahe, came up with his three laws of planetary motion (around the Sun). Ultimately, Sir Isaac Newton (1643 – 1727) established his force-based theory of gravity in his (1687) work Principia Mathematica in which the embedded rule of inverse squares ultimately explained Kepler’s third law of planetary motion. The changing paradigm was now complete. Its change began with Copernicus’ simple shift to the Sun as the center of planetary motion and ended with the description of gravity.
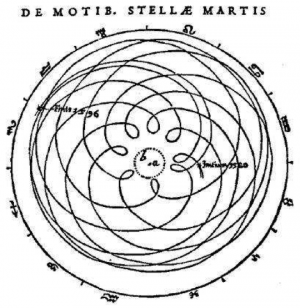
Big Bang Theory
Edwin Powell Hubble (1889 – 1953) an excellent high school and college athlete was a student of math and astronomy at the University of Chicago. He was granted an early Rhodes scholarship at the Queens College, Oxford becoming a student of jurisprudence, literature and Spanish. He later earned a PhD from the Yerkes Observatory, University of Chicago, in astronomy where he initiated his life’s work with his graduate dissertation “Photographic Investigations of Faint Nebulae”.
Hubble, after the War, began his work in astronomy at Mt. Wilson’s Observatory. Using Hooker 100 inch telescope, the world’s largest, he began his exploration of several spiral nebulae. Nebulae were considered to be formations within the Milky Way galaxy. The most accepted view of the cosmos was that the Milky Way was the totality of the Universe.
At the time of Hubble’s initial investigations, it was not known that there were two entirely different sources of nebulae. Many observed objects could only be within the confines of the Milky Way. These are composed of ionized gasses of either star systems in formation or remnants of star deaths. Edwin Hubble was drawn to another category of nebulae. Certain spiral formations showed themselves to be of a different origin. He focused on stars within these structures that were known as Cepheid Variables. Cepheids are large stars that oscillate like a pendulum from larger radii to smaller. These oscillations occur with a precise regularity. The periodicity is closely linked to the brightness of the star. For this reason they have become reliable “standard candles”. Since luminosity is known for Cepheids of a given period, the distance to these objects can be accurately determined by their apparent brightness.
Edwin Hubble in 1922 and 1923 observed Cepheid standard candles in several spiral nebulae including Andromeda Nebula and Triangulum. The Cepheids within these nebulae clearly were too far away to be part of the Milky Way. So the island universe theory fell by the weight of these proofs. These nebulae were in themselves galaxies.
The spectrum of light from an astronomical object shifts from its original frequency when that object is either approaching or receding from the observer. This change in frequency is known as a Doppler shift. Approaching astronomical sources have their spectrum shifted to the blue (shorter wavelengths) end of the spectrum while the light from receding sources is shifted to the red part of the spectrum (redshift).
Over the next several years data was gathered by Milton Humason and Vesto Slipher on Cepheids within 46 galaxies where the distance to that galaxy had been estimated. When Hubble plotted the distances with redshift, the greater the distance to the galaxy, the greater was the redshift. The higher redshifts meant these objects were receding from us faster than the lower shifts. In 1929 Hubble established his redshift distance law and with it Hubble’s constant. Thus it was established that the Universe was expanding.
Georges Lemaître had two years before stated that the Universe was expanding, but it was the specificity of Hubble’s and Humason’s calculations that put scale to the expansion and led to wider acceptance of the expanding Universe. Einstein, who originally believed that the Universe was held in stasis by an unknown pushing force that he called the cosmological constant, in deference to these proofs, stated that his static universe was the “greatest mistake of his life.”
Fred Hoyle expounded a “Steady State Theory” where matter is continually generated as the distance between Galaxies increases. His theory was considered seriously for a number of years. Ironically, it was Fred Hoyle who named Lemaître’s and Hubble’s theory the “Big Bang Theory”.
Over the next several decades, the Big Bang theory has been challenged by other variations of a perpetual universe including an oscillating cosmos that contracts and then bounces. Conflicting theories have failed to explain deep space views of the early Universe. With the discovery of the cosmic background radiation from the primordial Universe, the Big Bang theory has come to be the accepted paradigm. However, this is not the end. Various unexplained aspects about the expansion have led to a series of ad hoc theories that have brought with them their own challenges.
Cosmological Crisis
Questions arose within the backdrop of the Big Bang theory. The Big Bang established the initial conditions for the Universe. From this base came the unfolding of particles described in the standard model. Within the early phases of the Big Bang the fundamental forces of nature delinked to form the electromagnetic, electro-weak and strong forces. How this happened and how these three unified with gravity remained an open question.
The momentum of the initial expansion and Einstein’s General Relativity seemed to explain most of the observed motion in the Universe until closer inspection showed there was a considerable deficit of mass. And in a seemingly opposite phenomena, the Universe has showed evidence that the expansion is actually accelerating.
Then there is the origin of the Big Bang itself and, on the other side of time, its ultimate fate. If there is this Universe, are there others?
Questions included why matter was not evenly matched with antimatter, and why the atomic valences led to atoms such as carbon that enabled such complex molecules to form and provide the structure of carbon-based life.
The movement of matter and energy determined the distribution of the galaxies. Why is the Universe isotropic? Yet, how did this initial evenness lead to the grouping of galaxies leaving vast regions of space virtually void of matter?
The Big Bang hypothesis seemed to leave these and other questions unanswered. Attempts have been made to clear up these ambiguities. Some of these have led to major theories.
The original simple Big Bang theory now has a patchwork triage theories attached to it as attempts to explain all that is seen. As in the days of the original challenges to Ptolemy’s geocentric theory, today there exists a cosmological crisis. The triage cosmology of string theory, M-theory, inflation theory, dark matter, exotic energy and multiverse theories combine to produce a Ptolemaic-like crisis. A Copernican-like solution is required.
String Theory
String theory was formulated to define the nature of fundamental particles. When first proposed in the late 1960s, it attempted to explain the strong nuclear force. The advent of quantum chromodynamics negated the string theory solution. However, the work done seemed to indicate that that the exploration of string theory could lead to the description of quantum gravity.
String theory gained new life as a possible explanation of the nature of bosons. Bosons are the conveyors of force. They include gauge bosons that through the exchange of virtual particles transmit forces. Photons are the carrier of electromagnetic force; W and Z bosons convey the weak force; gluons communicate the strong force; and gravitons are the theoretical conveyor of gravity. Bosons have spins of whole numbers while the other class of elementary particles called fermions have half-integer spins. Multiple bosons can also occupy the same quantum space while Wolfgang Pauli’s Exclusion Principle forbids such state cohabitation for fermions.
String theory expounds that the Universe is comprised of minute vibrating quantum strings. There are two types of strings. The open string has unattached ends. The closed strings attach to themselves leaving no loose ends. The frequency of the strings and the dimension in which they vibrate is thought to determine the structure of the particles and forces they form. Within these theoretical dimensions not only are the known particles of the standard model defined, but the elusive graviton is expected to be unveiled
A worldline is a convention that tracks the movement through space and time where vertical movement is movement in time and horizontal movement is movement in space. A photon travels at the velocity of light and thus has equal movement in space and time. It has a 45º worldline. An object that has no movement in space nevertheless moves in time will have a vertical worldline. In theory, open strings trace out a worldline that resembles a strip whose angle tracks its spacetime movement. Closed strings form tubes. If a closed string encounters another particle and combines with that particle, it will form a type of “Y” combining the two tubes.
String theory later evolved to explain the interaction of all particles not just bosons. It then was named superstring theory. There are five versions of superstring theory. These are IA, IIA, IIB, HO & HE. Recently these seemingly different versions of the theory have been seen as special cases of the same broader theory put forward as a tentative theory of everything.
The complicated mathematics of the super-symmetry of string theory required additional dimensions. These dimensions are rolled up into such small configurations that they are unlikely to ever be observable. What remains are the three spatial and one time dimension of our familiar universe. Initially, bosonic theory required the addition of 26 dimensions. Superstring requires ten independent dimensions.
There are the traditional three Euclidean spatial dimensions with the fourth time dimension and six other unseeable spatial dimensions. The unobservable dimensions are rolled up in what are referred to by some theorists as Calabi-Yau shapes. Unobservable, they are immune to investigation. Each rolled-up dimension becomes an adjustable parameter for various versions of the theory. As a consequence, there are millions of potential permutations for the theory’s universal solution.
String theory is fraught with complications. Super-symmetry particle partners are a necessary component of the theory. These have yet to be discovered in particle accelerators even though some of the energies necessary for the unveiling super-symmetry have been reached.
The structure of the theories is removed from the observable universe due to the size of the theoretical strings. Only anecdotal evidence can be sited to justify the underpinnings of the string theory.
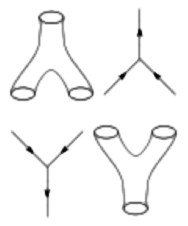
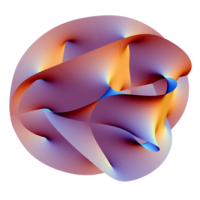
M-Theory
The five distinct string theories developed using different models for how strings are constructed presented a dilemma on which version provided the best solution. The five theories are considered to be special cases of a broader M-theory (standing for Membrane-theory) that seeks to unify these various forms. While the five theories require a ten dimensional structure, M-theory incorporates an eleventh dimension.
M-theory tries to add super-gravity to the five superstring modes. It describes the string as a one-dimensional slice of a two-dimensional membrane vibrating in eleven dimensions. The theory attempts to explain the universe as undergoing multiple incarnations when two parallel membranes within a ten-dimension universe separated by the seventh rolled-up dimension (eleventh overall dimension). These membranes periodically collide and generate another Big Bang. Multiple cycles of incarnation are thus generated as these membranes move apart and come together.
There is an ongoing effort to expand M-theories interpretation to explain “real-world” experience. The theory’s foundation and that for string theories is mathematical rather than a result of experimental evidence. There are actually a vast number of possible permutations within the unobservable dimensions of string theory and the broader M-theory. These adjustable parameters stretch the credibility of the theory and make it an extraordinarily complicated solution to the laws of the Universe.
Inflation Theory
Inflation theory developed by Alan Guth and Andrei Linde in the 1980s is an attempt to explain the homogeneity and isotropy of the Universe’s cosmic background radiation. It was felt that since remote portions of the universe could not communicate with each other considering that the fastest that a signal can be sent is the velocity of light, it would be impossible for the Universe to maintain such consistency of temperature in all directions. However, if the Universe in its earliest epochs (between 10-36 to 10-33 or 10-32 seconds) pushed by negative vacuum energy expanded at a fantastic rate greatly in excess of c, then the Cosmos that we see from our location in space would be a small proportion of the whole. This would make the Universe appear isotropic even if the entire structure is not.
Inflation is propelled by a type of vacuum energy that doesn’t abide by the limitations of Special Relativity. This out-pushing force happens without regard to the normal movement of time. It is not entirely clear what vacuum energy is and why the movement in space becomes divorced from the movement of time, but it has become the best candidate for the outward pushing force of inflation.
As seen from space the Earth is obviously a sphere, but when on a rowboat in the middle of a calm lake, the Earth looks flat. In this way, a tiny patch on any curved surface appears to be flat. So since inflation makes it so that we can only see a tiny portion of an inflated Universe the contours of that local vantage point make the cosmos appear isotropic.
Inflation tries to explain the evenness of the cosmos, but it is also put forward as a rationale for the uneven distribution of the Universe’s mass centers. There are large structures within the Universe. Some stretch over a billion light-years.
Massive galactic congregations, lengthy filaments connecting diverse mass centers, and vast voids make up the observable Universe. It is felt these structures originally were seeded by quantum fluctuations that grew to cosmic scale by the vast expansion associated with inflation.
What caused the inflation to end leads to additional speculation. It is assumed that the Universe exited the inflationary epoch slowing down to that predicted by the traditional Big Bang model through a random quantum perturbation called “bubble percolation”, which is conceptually similar to CO2 bubbles spontaneously forming in a newly opened bottle of soda. The random separation of our Universe from the inflationary cosmos left a rudimentary Universe. At this point the traditional Big Bang took form.
What is not known is what is expanding. Is it space itself? But isn’t space defined by the objects within that determine size? If matter didn’t form until after the inflationary epoch ended, then what gave dimension to the pre-Big Bang cosmos – vast empty space?
Dark Matter
When the rotations rates of galaxies were measured, it became clear that the velocities of the spiral arms exceeded that which can be accounted for by that galaxy’s apparent mass. Observations show that the mass required to produce the gravitational curvature necessary to generate the observed velocities of the stars within galaxies is several times that of the visible matter. The missing mass is assumed to be dark matter. Dark objects and black holes seem highly insufficient to make up the gravitational deficit.
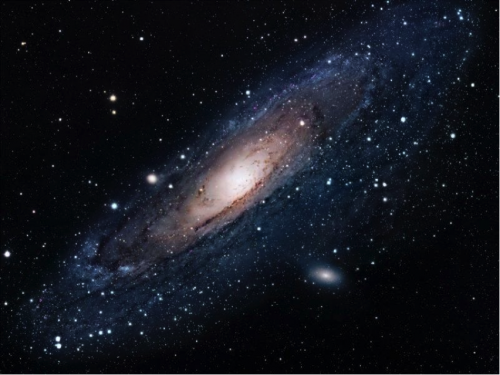
Dark matter theory assumes that the missing mass is from slow moving massive particles that do not interact with matter other than with their gravitational curvature. It is assumed that dark matter is made up with a new class of subatomic particles called WIMPs or weakly interacting massive particles. The problem with the WIMP solution is that the theoretical particles cannot be observed by standard measuring techniques that rely on the electromagnetic, weak or strong forces.
Particles are formed by the same three forces that WIMPs seem to evade. Therefore, an entirely new theory for particle formation must accompany any valid explanation of these elusive objects.
Since dark matter does not actively interact with itself, these theoretical particles should be attracted by other massive objects. They would most likely move in an oscillating fashion to and through these mass centers. The movement of these small but massive particles may be similar to the macro movement of spherical globular clusters. In this way they form a spherical halo within and around galaxies. The lens-like gravitation curves light from distant galaxies passing the more nearby galaxies on the way to Earth. The behavior of these gravitational lenses supports a spherical configuration for dark matter formations.
Abell 1689 is one of the most massive galaxy clusters known. The gravity of its trillions of stars, plus dark matter, acts like a 2-million light-year-wide “lens” in space. The gravitational lens bends and magnifies the light of galaxies far behind it1. Note the thin “stretched” galaxies on the circular perimeter around the brightest portions of the image. This is the lensing effect.
Dark matter is thought to represent 26.8% of the Universe’s mass-energy equivalence. Despite the compelling evidence for dark matter, virtually no progress has been made in identifying the theoretical particles thought to be responsible for the missing mass, nor has the standard model for particle formation been able to account for their structure.
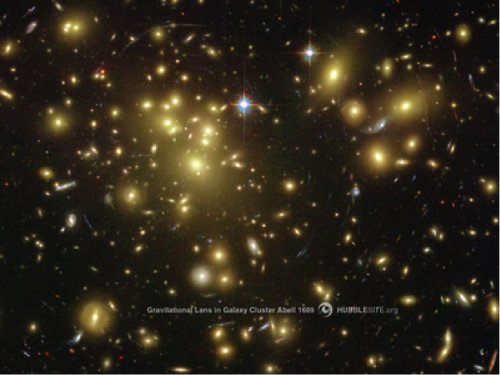
Exotic Energy
Distances to astronomical objects are determined by several methods. Nearby stars can be estimated by the change in viewing angle relative to distant background objects when viewed from one side of Earth’s orbit versus the other orbital extreme six months later. This is referred to as parallax. Since the diameter of Earth’s orbit is known to a high level of precision, the angle differential can be triangulated to an accurate distance in the same way that a surveyor judges distances. With the accuracy of the Hubble telescope, distances can be determined up to 10,000 light-years away. Beyond this relatively local perspective, other methods had to be developed to determine distances.
Brightness of objects varies with distance according to the law of inverse squares. In this way the distance to a 100 watt light bulb can be determined when observed in unobstructed space. The brightness of a one hundred watt bulb is known so proportionate illumination comparisons can be made. However, the brightness of astronomical objects must be known for the relative brightness of the object to be calculated. What were needed were objects of known brightness regardless of their distance. These objects became known as “standard candles”.
One of the most effective standard candles is a Cepheid variable star. A Cepheid varies from a larger brighter state to a smaller denser one. The classical Cepheids are large stars between four and twenty times the size of the Sun and up to 100,000 times more luminous. These stars oscillate in cycles from days to months. These yellow super-giants expand by millions of kilometers at their peak. The period of oscillation tells observers the intrinsic brightness of the object within a reasonable level of accuracy. The maximum distance calculations for Cepheids approximates 100 million light-years.
There are other standard candles, but the one that is effective up to the greatest distances is that of Type Ia supernova. Type II supernovae are super-giant stars that have exhausted their nuclear fuel and no longer produce enough energy through fusion to prevent the star’s gravitational collapse. These experience a catastrophic explosion that for a time can produce enough light to match the total luminous output of an entire galaxy. These supernovae have highly variable outputs depending upon the size and chemical makeup of the original stars.
Type Ia supernovae occur when a white dwarf and another less massive star orbit each other in close proximity within a binary system. The white dwarf pulls the outer atmosphere from its companion over time until it reaches the Chandrasekhar critical density. Originally, it was thought that gravitational pressure overwhelms the electron degeneracy pressure forcing the electrons to merge with the nuclear protons forming neutrons. When this occurs, the star implodes forming a supernova.
More recently, Type 1a theory states that the carbon-oxygen precursor white dwarf reaches 99% of the Chadrasekhar limit resulting in a flare from the Rayleigh-Taylor instability that leads to runaway fusion reactions resulting in a total unbinding of the star in just a few seconds.
Since every Type Ia is hydrogen poor and of approximately the same mass, i.e., 1.4 solar masses, all supernovae of this source produce near identical illumination, thus forming a perfect standard candle. The overwhelming brightness of these novae make them perfect long-distance standard candles. The distances measurable by the Type Ia exceed three billion light-years. More recently, supernovae methodology has approached a record ten billion light-years or ¾ of the distance to the Big Bang, as seen in the image on the next page of the Type II Supernova Wilson, SN UDS 10Wil.
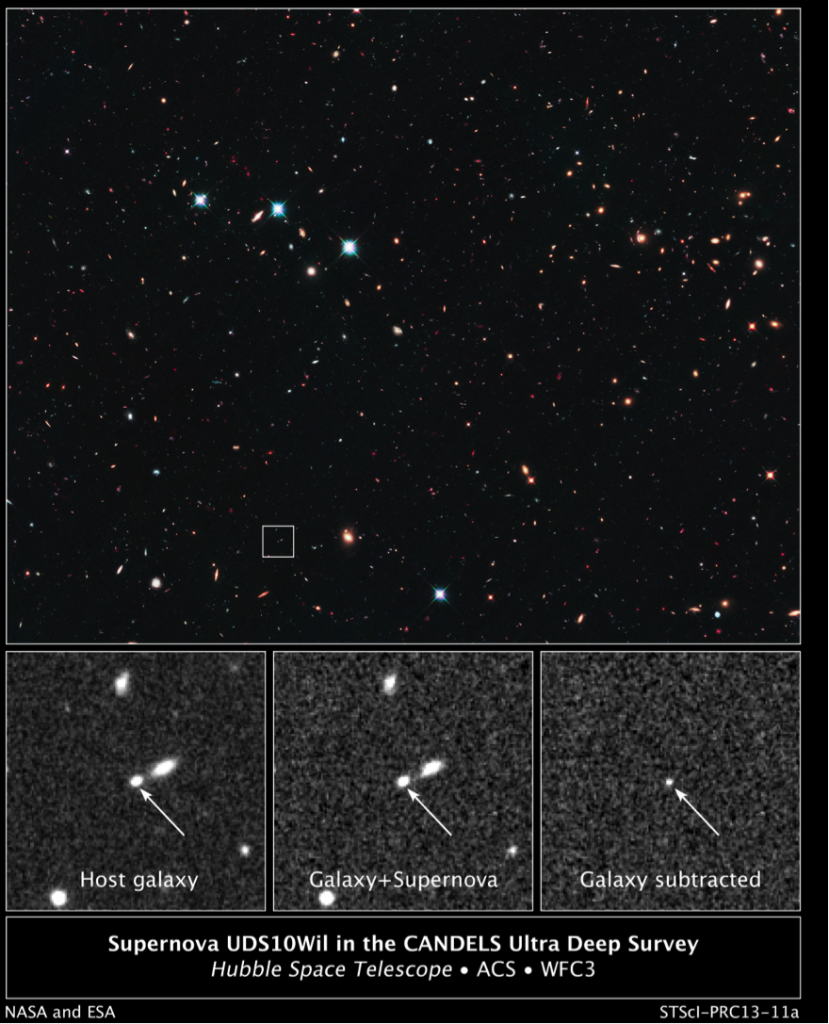
Edwin Hubble determined there was a relationship between distance and the recession velocities of galaxies. Recession velocities are determined by the Doppler shifting of the light from these objects. Certain wavelengths of light passing through a gas, such as hydrogen, are absorbed by the electrons in orbit around the nucleus. The wavelengths absorbed are specific to the energy differential between rest-state orbit and the new elevated energy orbit. Energy is also absorbed for electrons moving from one elevated state to a higher energy.
When light passes through a hydrogen cloud observed with a spectrometer, there appear dark lines at the energy level of the light, which absorb specific wavelengths. This generates a dark-line pattern that is hydrogen’s absorption signature.
The light emissions from approaching objects are blueshifted since the wavelengths are compressed by the movement of the light source. However, Hubble determined that virtually all distant objects are redshifted and realized that the Universe is expanding. The greater the distance to these objects the greater was the shifting of the light into the red area of the spectrum.
Redshifts (z) are a proportional calculation. They can be measured either by using wavelengths indicated by lambda (λ) or the frequency of the electromagnetic radiation (f).
Based on Wavelength
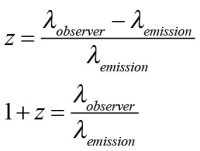
Based on Frequency
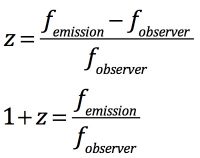
Blueshifts have z < 0 while redshifts have z > 0.
In the late 1990s type Ia supernovae were observed and comparisons were made of 1) their recession velocities from here as measured by the red shifting of the light from its original frequency, and 2) the distance of the objects as determined by the brightness of these “standard candles”.
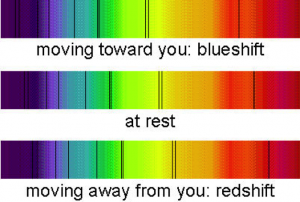
The Super Nova Research team composed of an international team of scientists headed by Paul Perlmutter at Lawrence Berkley National Laboratory indicated that the most distant of these objects are dimmer than it was assumed would be as determined by the redshifts. This implied that the Universe is actually accelerating, a result that was contrary to the then-existing paradigm. These conclusions were supported by the High-Z Supernova Search Team formed by Brian P. Schmidt and Nicholas B. Suntzeff.
It had long been debated whether the Universe was closed, flat or open. The research from these teams was directed to answer this cosmological question. Therefore, it was a total surprise that their observations indicated that not only was the Universe open but that it was accelerating.
A closed universe is one where the expansion rate cannot counter gravity’s attractive force causing the universe to reach a limit to its expansion and collapse on itself resulting in the Big Crunch, which is the reverse of the Big Bang.
An open universe is where the rate of expansion has sufficient velocity to overcome the attractive force of gravity. This would be similar to a space probe launched with enough velocity to escape Earth’s gravity.
The third option is that the universe is flat. A flat universe would expand at the exact escape velocity, i.e., with no excess velocity. Most scientists considered a flat Universe to be an unlikely result since that would mean that the Universe was expanding at the precise velocity that matches the mass-energy contained within its boundaries, which is an outcome that a random Universe would not tolerate.
Whether the Universe is closed, open or flat is determined by the mass-density of the Universe relative to its velocity of expansion. The density parameter Ω is used to symbolize mass-density.
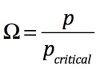
pcritical is the mass-density for a flat universe.
If Ω is greater than 1, then spacetime is considered spherical and the universe is closed, i.e., it curves back on itself. A triangle of sufficient size on its surface would have angles that total more than 180º as it would on Earth. In an open universe spacetime is portrayed as a saddle formation, which is hyperbolic. Here a triangle’s angles would total less than 180º. This universe would expand forever. For a flat universe spacetime is a plane. The summation of the spacetime angles for this scenario equals 180º.
The conclusions reached by cosmologists after evaluating the supernova Ia studies was that the Universe is not only open but that it is accelerating. This meant that some unknown force must exist that pushes space outward. When the Universe was smaller, the mass-density was great enough for the Universe to decelerate as in the typical Big Bang scenario. As the mass density declined, some pervasive pushing force caused the rate of separation between galaxies to accelerate.
The required force for the acceleration resulted in a new exotic (or dark) energy. There are two lead theories for the source of the expansion energy. One is a revision of Einstein’s cosmological constant. Albert Einstein proposed that for the Universe to be static, the predominant perception of that time, there must be some outward pushing force to offset gravity. He labeled this his cosmological constant. He later abandoned this concept for the expanding Universe described by Hubble’s model.
There is another theory called quintessence. Quintessence is dynamic in that it can be positive or negative. While the cosmological constant is just that – a constant, quintessence theorizes a changing force over time depending on the ratio of kinetic and potential energy. Proponents of this view believe that its expression switched from being attractive to repulsive about ten billion years ago.
One of the sacrosanct laws of physics is the conservation of energy. Energy may change from one kind to another or from potential to kinetic, but energy can not be created or destroyed. Exotic energy begs the question whether it is being created as the Universe expands or was there at the Universe’s formation? Is this energy included within the rest of the Universe’s normal matter and energy?
Little is understood about what this exotic or dark energy could be. Despite the lack of a theoretical base, it is now assumed that 68.3% of the Universe is comprised of dark energy, 26.8% is dark matter and the remaining 4% is traditional matter and energy. This leaves 96% of the Universe unexplained and unobservable.
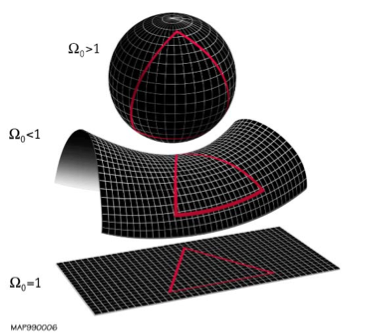
Multiverse Theory
Quantum mechanics has established that randomness is embedded in the event-creation process. Werner Heisenberg with his uncertainty principle concluded that the more accurately that you can determine the location of a subatomic particle, the less you know its momentum, and the more accurately that you can establish the momentum of the particle, the less that is known about that particle’s location.
It has been hypothesized that when given options for two events that one is expressed in this universe and the other is witnessed in identical universe except for that new event. So if you go to a street corner and turn right in this universe; in the parallel universe you go left.
There are obvious complications to the theory. Not only can you go left. You can go at any of the infinite possible angles to the right, left, forward or backwards; you can trip; you can yawn while going left, you can leap off of the curb; you can skip; etc.
This theory has been named multiverse. It is the ultimate attempt to merge quantum mechanics with cosmology. The most extreme case of multiverse theory is that every possible alternative to an action is realized in a different universe. In every quantum instant all possible courses both locally and over this vast Universe are realized in a new universe created around each possible outcome – no matter how unlikely. Every time that an electron randomly chooses one pathway versus the infinite number of other pathways, it results in not only the chosen pathway taken in our Universe but all the other possible pathways for new universes with the same initial set of conditions of our Universe. This results in independent universes for each possible pathway for the electron during each wave and for all particles and electromagnetic waveforms in the Universe throughout history.
Does It Make Any Sense?
The extrema versions of Multiverse theory are obviously patently absurd to the extreme, but each of the other theories advance concepts that cannot be tested. The velocity of light speed limit of Einstein’s theory of Special Relativity is blithely thrown out for the hyper-expansion of inflation theory. This occurred before observations existed leaving no direct telltale evidence. Dark matter and exotic energy conveniently are generated by unobservable particles or forces, but scientists have had no difficulty in allocating 96% of the stuff of the Universe to these immeasurables. String theories solve for inconsistencies by creating unobservable dimensions. It seems that modern interpretation of the uncertainty within quantum mechanics states that anything goes.
But what becomes apparent when one stands back and looks at these theories collectively is there is not a common thread or compatibility. Fundamentally, it can be asked, “Does it make any sense?” Theorists have replaced the search for elegant simplicity with abstract mind games and mathematical constructs.
There are more things in heaven and earth, Horatio,
Than are dreamt of in your philosophy.
— Hamlet (1.5.167-8), Hamlet to Horatio